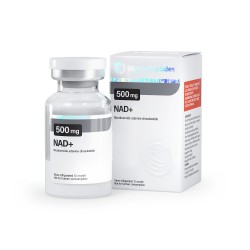
Ultima-NAD+ - Ultima Pharmaceuticals - US
MANUFACTURER Ultima Pharmaceuticals - US
WAREHOUSE USA Warehouse 5
SUBSTANCE
Nicotinamide Adenine Dinucleotide,
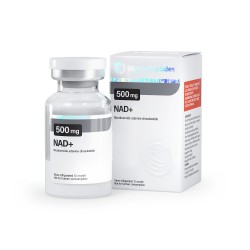
Ultima-NAD+
The problem is that as we age, we see a gradual decline in cellular NAD⁺ levels. This decline in NAD⁺ is linked to numerous age-associated diseases, including cognitive decline, cancer, metabolic disease, sarcopenia (age-related loss of muscle mass and strength), and frailty. Many of these diseases can be slowed down or even reversed by restoring NAD⁺ levels.
So, what exactly is NAD⁺, how is it made, and how is it used by our cells? Answering these questions will help us understand the roles of NAD⁺ in maintaining healthy aging and whether and how NAD⁺ deficiencies can be treated.
Where is NAD⁺ Found in the Cell?
NAD⁺ is compartmentalized into different structures within each cell. It’s found in the gelatinous liquid that fills the cell (cytoplasm), the cell’s battery packs (mitochondria), and where the cell houses genetic information (nucleus). These subcellular pools of NAD⁺ are regulated independently of each other, and consistent with this, the enzymes involved in the biosynthesis or degradation of NAD⁺ are highly compartmentalized as well.
How do Cells Make NAD⁺?
NAD⁺ mediates multiple major biological processes and is always in high demand. NAD⁺ is constantly synthesized, metabolized, and recycled in the cell to maintain stable intracellular NAD⁺ levels. Some cells, mostly in the liver, can synthesize NAD⁺ from scratch (de novo) using multiple dietary sources. NAD⁺ can be made de novo from L-tryptophan or vitamin precursors, such as nicotinic acid (NA).
Outside the liver, most cells do not express the full array of enzymes necessary to convert tryptophan to NAD⁺. But it can also be recycled or salvaged from nicotinamide (NAM), a by-product of when enzymes use NAD⁺ as a cofactor. Most tryptophan is metabolized to NAM in the liver, where it is released into the serum, taken up by peripheral cells, and eventually converted to NAD⁺. In this pathway, NAM is converted to nicotinamide mononucleotide (NMN) by an enzyme called NAMPT, which can be converted to NAD⁺. NMN also forms from nicotinamide riboside (NR).
What Enzymes Consume NAD⁺?
NAD+ is also continually turned over by three classes of NAD+-consuming enzymes: sirtuins, PARPs, and the NAD+ glycohydrolases — also referred to as NADases (CD38, CD157, and SARM1).
Sirtuins
With efforts to understand how NAD⁺ impacts aging, sirtuins have emerged as key actors. Since their discovery, the sirtuins have received much attention because they regulate key metabolic processes, stress responses, and aging biology.
PARPs
The human PARP protein family is composed of 17 proteins. Briefly, the PARP-mediated cleavage of NAD⁺ produces NAM and ADP-ribose as by-products. Overall, targeting PARPs, in particular PARP1, is a promising therapeutic strategy in the aging field. However, more studies are needed to fully understand the contribution of PARPs to the age-related decline in NAD⁺ levels.
NADases
CD38 and CD157 are multifunctional ectoenzymes — enzymes found outside or on the outer membrane of a cell. CD38 modulates essential cell processes, such as immune cell activation, survival, and metabolism. Importantly, other than NAD⁺, NMN is emerging as an alternative substrate of CD38, whereas CD157 consumes NR as an alternative substrate. Very little is known about the purpose of CD157 enzymatic functions in cellular biology and aging. However, recent evidence shows that, like CD38, CD157 is upregulated in aging tissues and may have a role in aging-related diseases, such as rheumatoid arthritis and cancer.
SARM1 was only very recently assigned to the NADase family along with CD38 and CD157. SARM1-mediated NAD+ degradation plays a key role in axonal degeneration after axonal injury. SARM1 is primarily found in neurons and promotes neuronal morphogenesis and inflammation., but it is also found in certain immune cells, such as macrophages and T lymphocytes, and regulates their functions. SARM1 is emerging as a therapeutic target to prevent or ameliorate neurodegenerative diseases and traumatic brain injuries.
How Else is NAD⁺ Used in the Cell?
Beyond the main groups of enzymes that consume NAD⁺ discussed so far, NAD⁺ is widely used as a cofactor or substrate for biochemical reactions. Over 300 enzymes are known to rely on NAD⁺ for their activity. Accordingly, NAD⁺ is a mediator of key cellular functions and adaptation to metabolic needs. Some of these critical cellular processes include metabolic pathways, maintenance and repair of DNA to safeguard genomic stability, and autophagy — the cell’s recycling mechanism. Collectively, these functions are important for maintaining systemic health and balance. However, during aging, declining NAD⁺ levels can impinge on these processes and exacerbate aging-related diseases.
How is NAD⁺ Related to Aging?
During aging, NAD⁺ levels decline, and many enzymes associated with NAD⁺ degradation and biosynthesis are altered. Many of the cellular processes that influence or are influenced by aging — such as metabolic dysfunction, DNA repair failure, genomic instability, inflammation, cellular senescence, and neurodegeneration — are regulated by NAD⁺ levels. Also, this decline in NAD⁺ levels during aging has been linked to the development and progression of aging-related diseases, including atherosclerosis, arthritis, hypertension, cognitive decline, diabetes, and cancer.
How Can NAD⁺ Levels be Restored?
NAD⁺ levels can be somewhat restored with dietary precursors like NMN, NR, and NAM. Also, The cellular level of NAD⁺ can be increased either by stimulation or activation of enzymes involved in NAD⁺ biosynthesis like NAD⁺ precursors or by inhibition of enzymes that consume or degrade NAD+. For example, targeting CD38 and CD157 with small-molecule inhibitors may make these commonly used NAD⁺ precursor metabolites more efficient in restoring NAD⁺ levels in aging individuals.
Some potential strategies that boost NAD⁺ levels include lifestyle changes, such as increasing exercise, reducing caloric intake, eating a healthy diet, and following a consistent daily circadian rhythm pattern by conforming to healthy sleeping habits and mealtimes.
There is a lot of research that has been conducted on the topic of circadian rhythm and sleep patterns. The circadian rhythm is the natural body clock that governs the sleep-wake cycle. This rhythm is largely controlled by the brain’s suprachiasmatic nucleus (SCN), which is located in the hypothalamus. The SCN is responsible for sending signals to the body that help to regulate the circadian rhythm.
The body’s natural circadian rhythm is what helps to dictate when we feel tired and when we feel alert. The circadian rhythm can be affected by a variety of factors, including our environment, diet, and exercise habits. It is also affected by our natural body clock, or chronotype. Our chronotype is what determines our natural sleep-wake cycle.
All of these approaches promote tissue NAD⁺ levels and are beneficial for health. These include improved tissue and organ function, protection from cognitive decline, improved metabolic health, reduced inflammation, and increased physiological benefits, such as increased physical activity, which may collectively extend patient healthspan and potentially lifespan.